Why MASW seismic methods for geology, seismic site classification, voids, sinkholes, fill, and anomalies
MASW seismic methods have grown increasingly popular among engineers and geologists because of the need to measure shear wave velocities at varying depths. Geologists use shear wave analysis to characterize soils and bedrock conditions. However, for engineers to understand the mechanical properties of soils and bedrock at depth, they depend on reasonable estimates of shear wave velocities to calculate Poisson’s Ratio, Young’s Modulus, and Shear Modulus. Until now, professionals relied on soil sampling and seismic methods that directly measured shear wave velocities. These approaches required boreholes and near surface geophysical methods that were not always user friendly. Today, we find that by analyzing the propagation of Rayleigh waves (e.g. MASW and ReMi) we can reasonably estimate shear wave velocities at depth without having to use intrusive borehole methods. Thus, the analysis of surface waves is an effective method for estimating the stiffness of the host material.
The benefits of MASW methods over conventional methods are numerous. It is a non-invasive, cost-effective technique that does not require boreholes and can be used in nearly all types of terrain. More importantly, it allows geologists and engineers to estimate shear wave velocities with no direct measurements. MASW and ReMi (Refraction Microtremor) are seismic methods that use the propagation of Rayleigh waves to estimate shear wave velocities at depth. This information allows geologists to characterize soils and bedrock conditions, as well as provide engineers with the data necessary for the calculation of Poisson’s Ratio, Young’s Modulus, and Shear Modulus. MASW can be used for a variety of purposes including: geologic mapping; seismic site classification; voids, epikarst, and sinkhole studies; and seismic surveys for geotechnical investigations. MASW is also beneficial in the detection of anomalies such as voids and fill that conventional seismic refraction methods may overlook.
How does MASW Seismic methods compare to other near surface geophysical methods
Seismic methods are based on the physical characteristics of subsurface materials, such as elasticity and density, in order to detect geological features beneath the surface. On the other hand, electrical and electromagnetic techniques (e.g., electrical resistivity imaging (ERI), electromagnetic terrain conductivity surveys (EM), or ground penetrating radar (GPR)) depend on the electrical properties of subsurface materials to detect features beneath the surface. Despite their different sources of energy, both seismic and electrical methods are effective in interpreting subsurface features.
Seismic techniques have the advantage of being able to detect changes in elasticity and density, while electrical methods can be used to identify materials with different unit resistivities or conductivities. For example, when mapping bedrock ERI conductivities increase with higher pore fluid conductivity and/or the presence of conductive clays. This can aid in identifying contamination or areas with increased secondary porosity due to fractures and faults. MASW, on the other hand, is not impacted by pore fluids, making its results independent of changes in electrical conductivity that affect ERI. By utilizing both methods together, more precise results and interpretations can often be achieved.
MASW Seismic Applications
Because Rayleigh waves are abundant, easily produced, and a major component of most seismic energy, there are numerous geophysical applications. Rayleigh waves travel along the Earth’s surface with a wavelength that increases with depth, making them ideal for geophysical applications. They have two common types of sources: active and passive microtremors. Passive energy-based surveys, such as those using naturally occurring microtremors, provide the most comprehensive understanding of deeper subsurface conditions, with the capability to reflect material properties at depths of hundreds of feet or more. In contrast, active source surveys, such as those using sledgehammers and plates, vibrators, weight drops, elastic wave generators, and other devices, offer greater resolution for shallow depths and produce cross-section type results.
MASW seismic surveys are used in geology, earthquake protection and engineering design, and geotechnical investigations (e.g., voids, epikarst features, sinkholes, and fill), locating voids, and locating anomalous zones of interest. One-dimensional (1D) passive or active/passive surveys provide a plot of shear wave velocities as a function of depth below a single location on the surface. Active survey results can also be supplemented with passive data, if needed. Two-dimensional (2D) cross-sections of results are easily created from active MASW surveys and multiple sections can be combined to form three-dimensional (3D) presentations. Finally, complex active surveys conducted with a true 3D MASW array yield detailed results of the subsurface conditions, which are determined through 3D algorithms.
Applications in Geology:
Since the early 1960s, Dorman & Ewing and other researchers discovered that inverting the velocity of Rayleigh waves (surface waves) could be used as an effective estimation of the shear wave velocity in a material. They also observed that Rayleigh wave velocities are roughly 0.92 times the shear wave velocity of a material. This breakthrough led to the development of methods that allowed professionals to map variations in soil and bedrock units using Rayleigh waves. Geophysically characterizing geologic conditions is in high demand. While many of the original concerns were about the effects of earthquakes, a global concern, the construction of a building, mapping the depth to bedrock for a solar farm, wind farm or quarry, or understanding the placement of geologic units around a landfill are local issues. Determining shear wave velocities of the subsurface in cross-section allows geologists, geophysicists, and engineers a method for mapping geologic units.
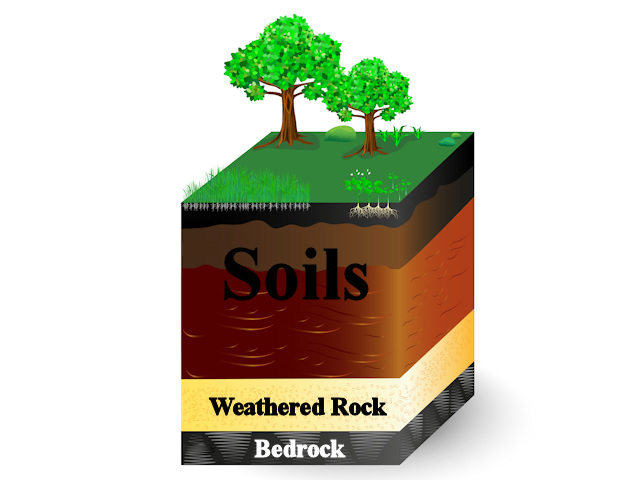
Rayleigh wave methods are often used to map geological units such as clays, sands, gravels, weathered rock and bedrock. These materials have a wide range of shear wave velocities from hundreds of feet per second to over five thousand feet per second, which helps to differentiate between soil layers at depth and/or bedrock. When compared to seismic refraction surveys, thin layers of water or perched water above bedrock do not adversely impact Rayleigh wave methods. Groundwater or fluids can lead to inaccurate depth estimates when using seismic refraction techniques as opposed to Rayleigh wave methods, such as MASW. Since the stiffness of the host material affects Rayleigh waves the most and fluids have minimal influence on the data, MASW seismic methods are a better choice for mapping geological soils and rock than seismic refraction methods. On the other hand, Rayleigh wave techniques are of little value for those looking to map groundwater or other subsurface fluids.
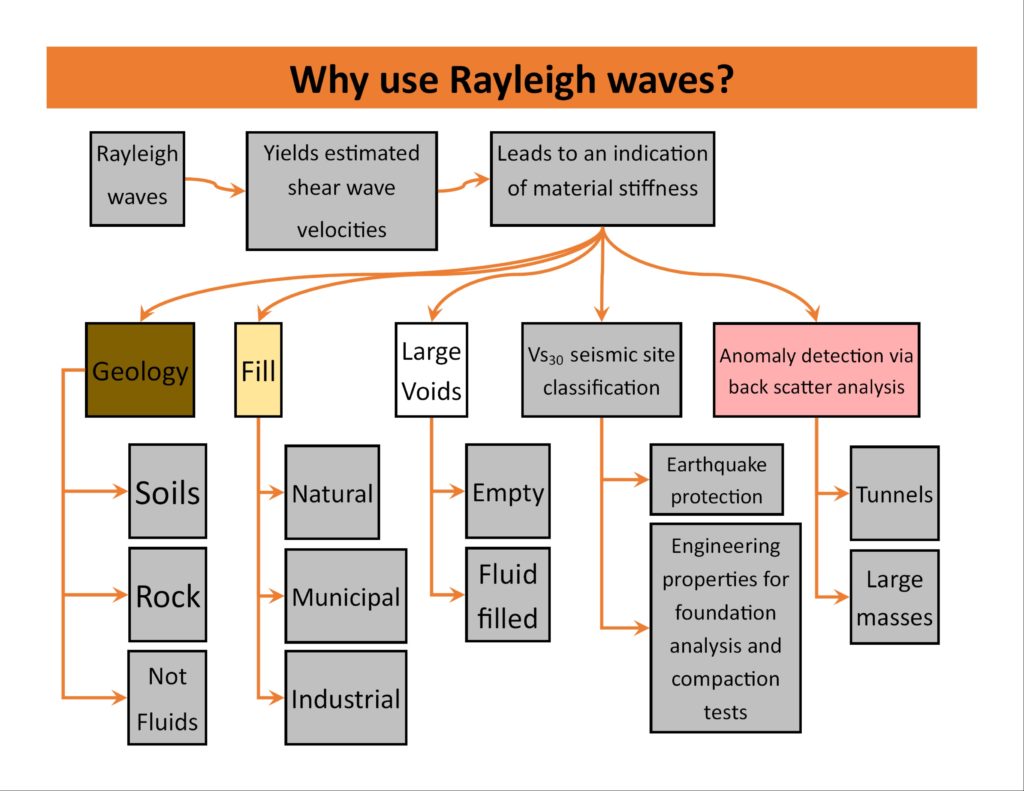
Applications for Earthquake Protection and Engineering Design:
One can use Rayleigh waves in the study of earthquakes, as they are one of the two types of seismic waves generated during an earthquake. The other type, known as body waves, originates from seismic energy that travels through the Earth’s interior. Rayleigh waves travel along the surface with a much lower frequency and greater wavelengths than body waves, making them easier to detect and measure. An earthquake can cause significant damage to buildings and other structures, particularly if the underlying soils cannot provide adequate support. Poorly compacted clays, for example, can cause foundation instability, resulting in cracking walls or even complete structural collapse. Similarly, unstable ground conditions can make it difficult to determine the best location for a building or structure.
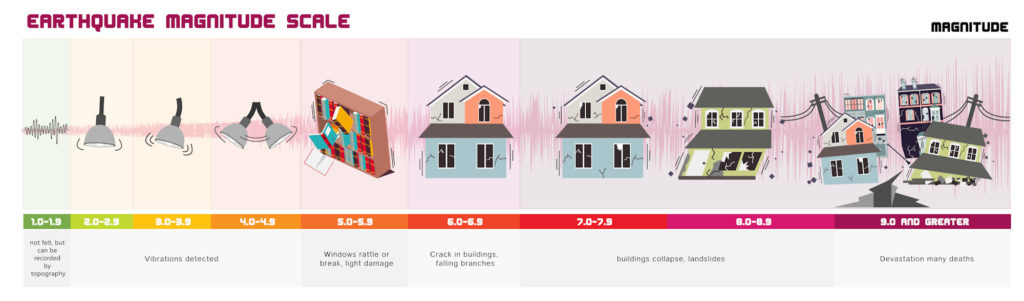
1-D Vs30 and Vs100 site soil classification
To reduce earthquake damage, geophysicists and engineers use Rayleigh wave surveys to characterize the stiffness and thickness of the soils. Thus, seismic site classification surveys estimate the average shear wave velocity to a depth of about 30 meters or 100 feet. Active and passive microtremor techniques are used to classify seismic sites based on shear wave velocities. The 1-D Vs30 Vs100 approach yields an average shear wave velocity that correlates to a NEHRP site classification, which helps determine the expected ground motions for different sites and can evaluate potential risks from earthquakes. Besides NEHRP, many depend on ground motion prediction equations to estimate the peak ground acceleration (PGA) that can be expected to occur at a site. The PGA values are then used to design structures that can withstand the shaking that is likely to occur in an earthquake. By utilizing Rayleigh wave surveys, seismic site classification surveys, active and passive microtremor techniques, and ground motion prediction equations, geophysicists and engineers can evaluate potential risks from earthquakes and design structures that are resistant to earthquake damage. This helps reduce the effects of seismic activity on people and property.
MASW 2-D and 3-D Seismic Surveys for Vs30 and Vs100 values
The two-dimensional MASW seismic survey is an effective way to verify subsurface conditions for earthquake studies. MASW produces a visual representation of the variations in shear wave velocity (Vs) across a specific area by creating colored cross-sections of Vs as a function of depth. This allows engineers and developers to gain insight as to where one may find the most suitable soils for building. Furthermore, MASW surveys offer an added bonus over traditional 1-D passive microtremor methods because they provide both cross-sections and Vs30 and Vs100 values, which classify the build area. When soil compaction is needed, one can compare the pre-compaction and post-compaction results to make sure that the work accomplished created the desired outcome. One can gain even more detailed insight into progress by conducting several MASW surveys throughout the compaction process. By using this technique it enables an engineer to carry out a broader base analysis to optimize foundation design.
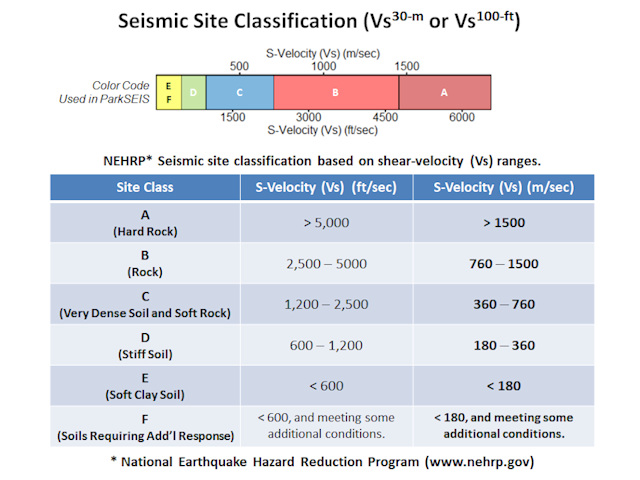
Applications in Geotechnical Investigations:
MASW seismic surveys in geotechnical investigations provide numerous advantages, enabling investigators to detect voids, map sinkholes, locate epikarst features, map the location of fill material, dynamic compaction, and assess the effectiveness of grouting operations.
Surface wave methods for locating voids, epikarst features, and sinkholes
Void detection, mapping sinkholes, and locating epikarst features is a complex and challenging task, requiring specialized techniques. Ground penetrating radar and electrical resistivity imaging (also known as electrical resistivity tomography or ERT) are two methods that are useful in certain subsurface environments. For ERI, soils or weathered rock that have high electrical conductivity with large air-filled voids create a significant electrical contrast, therefore, likely well-suited for detection. However, when the electrical conductivity of the subsurface is low with smaller air-filled voids, there may not be a sufficient electrical contrast for reliable ERI results. On the other hand, ERI and GPR may be an excellent choice in low conductivity materials, if there is clay infilling or the void spaces are filled with water. In the end, MASW is suitable because it is not affected by variations in electrical conductivity at depth.
Fortunately, Rayleigh wave methods present an alternative approach for detecting possible voids, epikarst activity, or sinkholes in any situation. MASW employs a group of multiple receivers that move along a transect line to identify dispersion characteristics that correlate well to these zones of interest. This technique is reliable as smaller wavelengths are sensitive towards shallower yet smaller features while longer wavelengths are better suited for larger features buried deeper down. MASW Backscatter Analysis (BSA), a lesser-known technique, using ParkSeis software filters out the background response to accentuate seismic energy that would otherwise have go unnoticed in unprocessed field results. Ultimately, MASW is incredibly efficient – because of its reliance on Rayleigh waves rather than electrical contrasts.
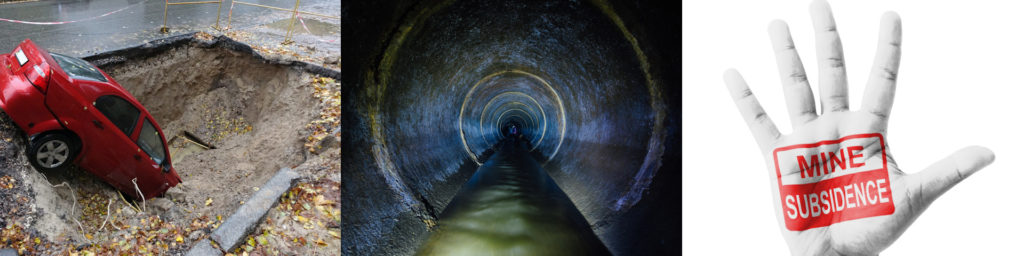
Surface wave methods to characterize fill and monitor dynamic compaction
Rayleigh wave methods are particularly well-suited to characterizing various types of fill, such as natural soils, municipal fill, and industrial fill. These types of fill often have different seismic characteristics than the in situ surrounding materials, making them easily distinguishable in the MASW results. For instance, the response of an abandoned quarry wall that has been covered with fill material can be remarkable and easily detected. Similarly, thick deposits of ash or slag can be mapped using MASW methods. These materials seldom have the same seismic properties as the in situ geologic units. By taking advantage of the contrast between undisturbed soils and the fill, one can map the boundaries. This makes an MASW seismic survey ideal for detecting fill and mapping the boundaries, which may not be visible at the surface.
Dynamic soil compaction is a process used to increase the density of soil through vibration and/or pressure. This compaction process is often used in construction projects to create a higher bearing capacity and make a firm foundation for structures. It also helps to reduce settlements and stabilize the soil, which can be especially important in areas with seismic activity. Monitoring dynamic soil compaction can be a challenging task for engineers due to the complexity of the process, as well as its great importance for a successful engineering project. To monitor the compaction process, engineers can employ MASW surveys to detect variations in the compaction or stiffness of subsurface materials. An MASW survey before and after compaction can monitor the changes made during a dynamic soil compaction program, enabling engineers to make well-informed decisions regarding their projects.
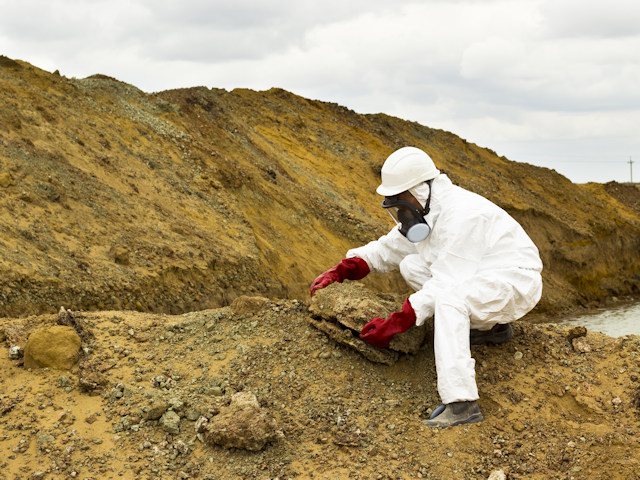
Surface wave methods to characterize grout
Several geophysical surveys may assist with assessing the effectiveness of grouting subsurface environments. The MASW seismic method is one approach, which yields shear wave velocities that can monitor a change in state associated with injecting or pumping grout. Park and colleagues have extensively explored how analyzing dispersion images before, during, and after grout injection can provide key insights into the efficiency of the grouting process. While the one-dimensional analysis may be sufficient under certain circumstances, a two-dimensional approach is typically recommended for more detailed monitoring. One can do this through a single two-dimensional MASW line or multiple lines at strategic locations. Occasionally, a true three-dimensional MASW solution may be the most suitable option. Without a doubt, a true three-dimensional survey is an arduous endeavor. However, an alternative solution is to use geo-referenced two-dimensional MASW lines in proximity to each other to generate a 2D-3D representation.
Applications in locating anomalous zones of interest
Up to this point, we have discussed applications associated with known targets or zones of interest. A broader class of Rayleigh wave applications are often used to locate anomalies, meaning areas that don’t match up with the surrounding environment or which stand out in a certain way. Anomalies can be buried foundations, underground bunkers and tanks, storm sewers, abnormalities in railroad ballast, and more. In order to analyze MASW data to locate anomalies, there are three common methods that can be utilized.
Typical MASW analysis
Like most of the discussion on this website, this approach uses the general MASW approach. Anomalies are revealed as distinct areas that contrast dramatically from their homogenous and isotropic surroundings. In other words, after processing the MASW data, one analyzes the results to locate any unexpected changes in velocities with respect to depth on standard MASW cross-sections. Inconsistencies or discontinuities may suggest a zone of interest or anomaly that may require further investigation.
MASW backscatter analysis or BSA
While BSA of MASW data is discussed above under void detection, this second method can apply to other potential sources. The general idea behind the BSA (Backscatter Analysis) approach of MASW data is to improve the response by enhancing the visibility of anomalies along a MASW line. Simply put, the primary benefit of the BSA approach is that it can detect and analyze subsurface features at greater depths than non-BSA MASW seismic results. Rayleigh waves, which are generated from seismic energy, are reflected off varying density and velocity contrasts in the subsurface. This results in the Rayleigh waves changing direction and character, creating a visible anomaly in BSA filtered seismic sections. By enhancing standard MASW results using BSA, one can identify anomalies along non-BSA MASW lines that could have otherwise gone undetected.
The analysis of common offset gathered Rayleigh wave data
The third and final method is to have ParkSeis MASW software sort the seismic data collected for the MASW approach into common-offset gathers, which are organized by the same source-to-trace distance. Common offset gathers are an important tool for the analysis of Rayleigh wave data, since they allow seismic energy to be observed with respect to its origin instead of simply viewing it as individual traces or stacked sections. This method allows one to observe any variations or anomalies existing in the seismic reflection data. By analyzing the common offset gathers of Rayleigh wave data, one can look for reflections that indicate changes in the subsurface. Unexpected reflected energy can indicate various geological features or objects, such as faults and buried structures. Through various filters and display settings, these areas can be identified more clearly. The analysis of Rayleigh wave data using common offset gathers can be a powerful tool for interpreting seismic reflection data and providing information about the subsurface structure.
A summary on why to use MASW seismic methods for geology, seismic site classification, voids, sinkholes, fill, and anomalies
Above are some of the many reasons to use MASW seismic methods. Using MASW seismic methods are becoming increasingly popular because of their wide array of applications and ease of use. They can help geophysicists better understand the subsurface world and the properties of various materials. Knowing the structure of the subsurface is an important factor in many areas, from engineering design to characterizing geology, seismic site classification, detecting voids or sinkholes, mapping epikarst activity, characterizing fill, monitoring the progress of dynamic soil compaction, and locating areas of interest or anomalies. With ongoing advances, MASW seismic methods will continue to be an invaluable tool for understanding our subsurface environment.
The benefits of using MASW are clear: it is a cost-effective, non-invasive seismic method that provides reliable data on shear wave velocities. With the help of modern computing power and advanced software, MASW seismic methods can quickly and precisely assess subsurface conditions.
Why MASW seismic methods for geology, seismic site classification, voids, sinkholes, fill, and anomalies
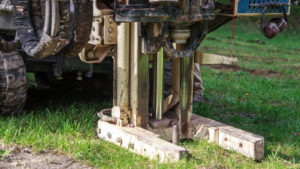
1-D, 2-D, and 3-D MASW surveys with active, passive, and combined sources, methods, and applications
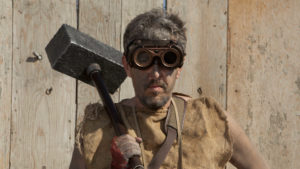
MASW seismic services, processing, equipment, rentals, and software
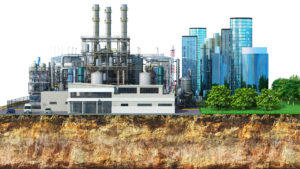
MASW case histories on mapping bedrock, mine reclamation, vibratory hammers
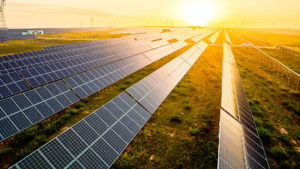
Special MASW content on theory, concepts, innovations, concerns, and credits
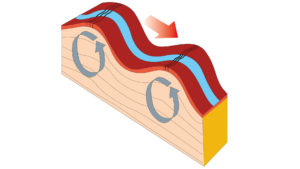
Contact MASWSeismic.com
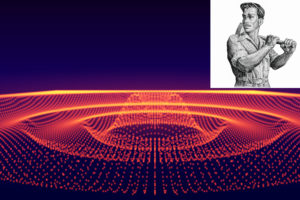